Northwestern Research Predicts New State of Matter
By Ed Finkel and Matt Golosinski
Anna Grassellino and James Sauls, Center for Applied Physics and Superconducting Technologies codirectors, hold a super-conducting RF cavity inside the Material Science Lab at the Department of Energy’s Fermi National Accelerator Laboratory. Photo by Reidar HahnFor Northwestern physicist Jim Sauls, the little things really matter in his quest to understand the strange nature of matter that’s normally hidden in everyday life. How strange? Imagine a cup of coffee where the java flows right through the bottom of the cup, while also crawling up the inside of the container.
Sauls’ investigations into the fundamental properties of matter and radiation are discovering exotic new phenomena that illuminate the differences between “classical” and “quantum” liquids and solids, research that may contribute to new superconductors and superfluids — frictionless fluids with very high heat conductivity. Their applications could be wide ranging: for instance, particle accelerators for probing nature’s fundamental forces, the development of better superconducting magnets for medical imaging, and superconducting devices for future quantum computers.
At typical room temperature and pressure, most materials present us with their familiar form as gas, liquid, insulator, or metal. In our everyday lives, the staggering number of randomly moving particles — the electrons in a metal, say — are subject to various effects that obscure the laws of the quantum physics. The properties of matter, particularly liquids, look bizarre when physicists push the bounds to see how matter behaves under extreme conditions of low temperature. For example, quantum materials such as UPt3 and liquid helium-3 (the lighter isotope of helium) undergo state changes and become superconductors or superfluids when cooled to low temperatures within fractions of a degree above absolute zero (minus 459.67 degrees Fahrenheit) something Sauls and collaborators Robert Regan, Bill Halperin and Keenan Avers achieved in two recent papers published in Physical Review and Nature Physics.
Helium is unique among all the elements in that it never freezes. When subjected to near absolute zero, helium atoms move into lower and lower energy states. As they get colder and colder, more of the atoms fall into the lowest energy state; they stop moving independently and instead begin moving together, in aggregate, exhibiting the fundamental quantum nature on a macroscopic scale. This is the superfluid state in which atoms move in unison.
Prof. Sauls makes superfluidity more familiar by using a transportation analogy. Imagine the helium particles as cars on an eight-lane interstate, he says. When the density of cars traveling gets large enough, small changes in speed occur to avoid collisions, leading to a slowdown of the overall flow, eventually resulting in gridlock. “That is like the viscous state of a normal liquid,” explains Sauls, who is on the executive committee of Northwestern's INQUIRE, a research institute that spurs cross-disciplinary investigation of quantum science. “But if we could install in each particle a communication device to lock its speed and distance to each of its neighbors so that the motion is coherent among all its neighbors, and so that this coherence applies to each and every particle, then the stream can flow bumper-to-bumper without collisions, slowing down, or friction.”
Understanding the collective behavior of quantum fluids and solids, says Sauls, is important to advance quantum physics and quantum materials science, and that research could eventually lead to new materials and techniques with profound importance for a variety of fields that can benefit from the quantum properties of superconductors, from medical diagnostics to quantum computing.
Indeed, superconductivity and superconducting materials are at the heart of low-temperature quantum technologies – from ultra-sensitive magnetometers called SQUIDS for mapping brain activity, to superconducting magnets used in MRI machines for medical diagnostics, to high-speed trains based on superconducting levitation and locomotion that may soon become commercial in Japan. And the high frontier, he says, may very well be ultra-sensitive superconducting devices for exploring fundamental physics and superconducting circuits for computers harnessing the computational power of quantum mechanics.
Collision Course
As co-director of the Center for Applied Physics and Superconducting Technologies (CAPST), a joint research venture between Northwestern and the Fermi National Accelerator Laboratory under the auspices of the University’s Office for Research, Sauls and his colleagues are engaged in a variety of investigations aimed at exploring matter’s fundamental nature. One significant research stream involves science that helps advance high energy particle accelerators — a tool that enables scientists to better understand the sub-atomic world, the forces governing the known fundamental particles, and to search for what is still unknown about much of the matter in the universe, ``dark matter.’’ Researchers in CAPST are at the forefront of understanding and developing technology for accelerating particles to the highest energies achievable. The technology is based on superconducting radio-frequency (SRF) cavities.
SRF technology generates electromagnetic forces that accelerate subatomic particles, like protons and anti-protons, so they collide at very high energies, with speeds near the ultimate speed of light, producing a beam of matter that creates huge amounts of energy in a confined space.
While some accelerators, like the well-known Hadron Supercollider at Fermilab in Batavia, Illinois, or the Large Hadron Collider near Geneva, Switzerland, have been used primarily for physics research (the discovery of the Higgs Boson, for example), many of the more than 30,000 particle accelerators in existence worldwide have applications for medicine, energy, national security, and other fields. CAPST researchers at Fermilab and Northwestern are among those pushing the boundaries of accelerator research. CAPST began about three years ago after a team at Fermilab led by physicist Anna Grassellino discovered a process to make the Niobium SRF cavities, the basic component of particle accelerators, more efficient and more powerful.
“That was a tremendous boost,” says Sauls. An expert in theoretical physics, Sauls uses statistical mechanics and quantum theory to investigate condensed matter, research into the “fundamental properties of matter and radiation.” “When you can use fewer, more efficient cavities for your accelerator, you save enormous costs. The amount of cryogenic cooling that you have to use in order to get them to operate goes down dramatically.”
Seeking a more systematic approach to further advance its particle accelerators Grassellino and Alex Romanenko at Fermilab contacted Sauls and his colleagues for assistance in determining how the theory of superconductivity and materials science could be brought to bear on behalf of the SRF cavity and overall accelerator performance.
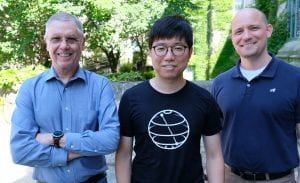
With a team of about 30 researchers, CAPST explores both the SRF cavities themselves and also how to develop novel superconducting materials with potentially new functionalities in electronics. Sauls describes such materials as existing in “a state of matter in which the entire system becomes like a giant atom … so that an electrical current, rather than being degraded by the scattering off of imperfections in the metal, or off of the edges and boundaries, the electrons just flow completely, without resistance.” This gives the SRF cavities their powerful surge.
Another area of CAPST research involves the development of superconducting technologies, mainly focused on the basic elements of quantum computers, known as quantum bits, or ``qubits.’’ “This is an area of active research, and it’s one that the government has decided to invest heavily into over the next decade by creating national centers for quantum information science,” Sauls says. “Superconducting qubits and microwave cavities are the basis for the current technology used by Google, IBM, Rigetti Computing, and labs all over the world. Part of Northwestern’s strength in this area includes my colleague in CAPST, Jens Koch, associate professor in Physics and Astronomy, who is one of the leaders in the field of superconducting-based quantum computing.’’ This is a rapidly developing field and Northwestern’s strengths in superconducting technologies, materials science and devices for sensing and precision measurement will provide new research opportunities enabled by the National Quantum Initiative.
Helium ‘Tornadoes’ Spin New Theories of Quantum Matter
Among the recent CAPST research projects that Sauls finds particularly exciting is his collaboration with PhD student Robert Charles Regan. They explored the nature of the phases of matter produced as a variant of helium which liquefies, but does not freeze even as it cooled to the temperatures approaching zero degrees Kelvin (absolute zero).
Their new research paper on “New Quantum Phases of Matter Confined in Quantized Vortices” considers the states of matter that exist in the light isotope of helium, called helium-3, which has one fewer neutron that the prevalent form of helium. Regan and Sauls describe how liquid helium-3, which forms a superfluid at low temperatures, behaves dramatically different than well-known liquids like water. Superfluid helium-3 remains at rest even as a confining vessel is rotated — at least up to a critical rotation speed. As the chamber rotates more quickly, helium-3 eventually begins to rotate, and as it does so ``quantized vortices’’ — which resemble tiny “tornadoes” — begin to form within the fluid. These vortices represent the discrete quantum nature of helium-3. Liquid helium-3 can rotate only with discrete values of angular momentum in integer multiples of Planck’s fundamental quantum unit.
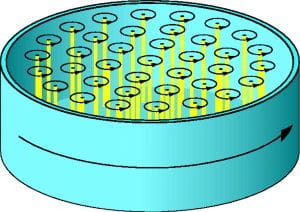
Similar to tornadoes and hurricanes, quantized vortices possess an ``eye’’ – the core of the vortex – in which the velocity drops dramatically. The low-pressure environment inside the eye is far from the violent storm outside the eye. The new report on the states of matter in rotating helium-3 shows that the ``eyes of quantum vortices’’ possess new states of matter. These are large regions of space, again, analogous to the hurricane’s eye.
The lowest energy state, called the ground state, is where atoms “prefer” to be as long as they are not “perturbed,” Sauls says. But once the chamber begins rotating sufficiently fast, “They can be in a multiplicity of possible states,” he says. “You have whole regions in the chamber where you have new quantum phases of matter.”
“When we cool down to very low temperatures — two millikelvin — we identify two different tornadoes, their regions of stability, and their properties,” Regan adds. “They look different; they act differently. One lives at a little lower temperature and higher pressure. We really nailed down the stability of these two microscopic tornadoes that live in a fluid near zero Kelvin.” These new states of matter form within the low-velocity depressions of the cores of the vortices, which Sauls compares to new types of plants that might grow where a forest fire has raged
Regan’s research analyzed and characterized the states of matter within those vortices, as well as predicting the boundaries between them. “Robert’s contribution was really nailing down which of these phases confined within the quantized vortices were stable at different temperatures, pressures, and magnetic fields,” Sauls says. “And then also elucidating the nature of the matter that grows in the region of confinement inside the eyes of the tornadoes.” Several confined quantum phases are stabilized depending on pressure, temperature, and magnetic field, including liquid crystalline phases of oriented molecular helium-3, ferromagnetic helium-3, and a uniquely quantum phase of chiral diatomic molecules distinct from polyatomic chiral enantiomers familiar in biochemistry. The results also confirmed the predictive power of the theoretical tools developed by Sauls and his research group to understand the nature of quantum phases with many competing ground states.
The study is at the forefront of experimental research in quantum materials, and specifically in quantum liquids and superconductors, which is the role of confinement and its interplay with phases of matter described by two key principals of modern theoretical physics: quantum states of matter related to the breaking of symmetries of the fundamental interactions between elementary particles, and the role of topology in quantum matter.
The role of confinement of quantum phases is also central to SRF accelerator technology. ``The ability to predict the behavior of the superconducting phase within the 100-nanometer region of confinement of the supercurrents in SRF cavities is essential to the advancement of the technology for both particle accelerators as well as quantum sensing and quantum computing applications,’’ Sauls says. “The operation of these massive particle accelerators depends upon the physics that happens within the 100-nanometer region of the vacuum superconductor interface. When superconductivity breaks down, the whole system collapses. So understanding the effects of confinement on quantum phases of matter is at the heart of a lot of the applications.” What is interesting to pursue going forward is to understand the mechanism responsible for breakdown. ``Most probably breakdown occurs by the proliferation of quantized vortices. When these objects move, they dissipate energy and produce heat,’’ Sauls says. “Understanding the structure and nucleation of these vortices has important implications for how to suppress dissipation from vortex motion. This is the direction our research is heading.’’
The researchers also note that a deeper understanding the physics of superfluids has implications for other scientific pursuits, including astronomy. For instance, it’s thought, based on observations of pulsars and extensive theoretical studies, that superfluids make up the interiors of dense, compact stars made mostly of neutrons (``neutron stars’’), which are observed as pulsars. Beyond the matter inside neutron stars, one candidate for the unseen “dark matter” in the universe is a quantum state of light-mass particles that forms a cosmic superfluid. The search for dark matter is a major physics enterprise and a key research direction by faculty in Physics and Astronomy and CAPST researchers at Fermilab.
On a more local level, the field of table-top atomic physics was dramatically changed by the discovery of low-temperature atomic clouds of atoms whose properties are dominated by quantum physics and superfluidity of the atoms, results which confirmed the prediction of a quantum phase of matter — now understood to be a superfluid — by Albert Einstein in 1925.
“It is a remarkable fact that the basic mechanism of macroscopic occupation of a single quantum state by the atoms of a cold atomic gas (the superfluid state) predicted by Einstein in 1925, and his 1935 paper with Podolsky and Rosen highlighting one of the fundamental features of quantum mechanics — entanglement — are two key concepts at the heart of a technological revolution before us in quantum information science,” says Sauls.